
1 July 2021
Marie-Valentine Florin
Given the urgency of deploying all possible ways to combat climate change, and in light of lessons learned from the Covid-19 pandemic outbreak that it was a mistake to ignore signals and not prepare for worst-case scenarios, this article suggests that techniques for removing CO2 from the atmosphere and sequestering it as permanently as possible should be deployed. It also recommends that more consideration be given to research and governance of techniques for reducing warming. Preparation is not a commitment to implementation. And failing to prepare because these techniques involve risks is a mistake. This piece elaborates on IRGC’s report on governance issues related to climate engineering. The report, published in 2020, is a thorough review of techniques and associated potentials, risks and uncertainties, and governance mechanisms. In the months ahead, other articles will focus on key aspects to implement risk- and evidence-based strategies to complement GHG emission reduction and climate adaptation.
Emissions reductions alone will not achieve the Paris goals
The evidence is mounting that current efforts to mitigate climate change will not be enough to achieve the climate goal set by the Paris Agreement of limiting global warming to well below 2°C, and preferably to 1.5°C, compared to pre-industrial levels. A growing number of institutions have been publishing authoritative analyses that should act as a wake-up call and spur greater action on complementary strategies. Among the most prominent bodies that have warned of the need to go beyond emissions reduction is the Intergovernmental Panel on Climate Change (IPCC), notably in its special report on global warming of 1.5°C, and the same message is expected to be repeated in the forthcoming Sixth Assessment Report. Other institutions that have sounded the alarm include the US National Academy of Sciences on climate interventions in 2015, on negative emission technologies in 2019 and solar geoengineering in 2021, and the UK Royal Society in 2018. Also of note is a 2019 Swiss government initiative to raise this issue at the UN Environment Assembly, and other national strategies towards deployment of negative emission technologies.
None of this is to ignore the increasingly aggressive commitments that governments and businesses have been making to decarbonise the economy. However, despite these efforts, the evidence suggests that some intentional large-scale human interference in the earth system will be needed. In this article, we argue that climate engineering warrants greater attention and that it is becoming increasingly urgent for policymakers to develop a full portfolio approach to climate change responses.
What is climate engineering?
Climate engineering refers to a set of technologies that deliberately alter the climate and can be used to partially offset climate change or some of its impacts. There are two categories of climate engineering technologies: carbon dioxide removal (CDR) and solar radiation modification (SRM).
CDR (also referred to as negative emission technologies, NETs) seeks to reduce the concentration of carbon dioxide (CO2) in the atmosphere. Although it does not help with the anthropogenic root cause (unsustainable economic, industrial and consumption practices), it does help with the source of climate change (greenhouse gases) and reduces its impacts, including warming, extreme weather events and ocean acidification. The term covers a variety of techniques that reduce atmospheric CO2 in different ways. The main examples are:
- Nature-based CDR, which typically traps CO2 using biomass such as soil or trees, or through alkalinisation of the oceans
- Hybrid methods, such as bioenergy with carbon capture and storage (BECCS), which involves the combustion of biomass for energy with the resulting emissions then being captured and sequestered
- Engineered CDR, including direct air carbon capture and sequestration (DACCS), which involves removing CO2 directly from the air and sequestering it.
While DACCS is expensive, it is among the most interesting techniques because, unlike the others, there is, in principle, no limit to the amount of CO2 that could be removed from the atmosphere. However, after being removed, CO2 must be sequestered, and it is not clear how this can be achieved on a large scale, and permanency can be an issue.
The second group of climate engineering technologies, SRM (also referred to as solar climate engineering or solar geoengineering), does not affect the level of CO2 or other greenhouse gasses in the atmosphere. Instead, SRM moderates warming by increasing the amount of sunlight that the atmosphere reflects back to space or by reducing the trapping of outgoing thermal radiation. Therefore, it addresses only one consequence of climate change, temperature increase. While SRM could thus help offset extreme weather events caused by that warming, it would not affect other impacts such as ocean acidification.
- One prominent SRM technique is stratospheric aerosol injection (SAI), which involves injecting fine aerosols into the high atmosphere to reflect more sunlight away from the earth. We have examples of how past volcanic eruptions have been followed by a period of cold weather, but SAI techniques are still immature.
- Other examples of SRM include marine cloud brightening (MCB), which also seeks to reflect more sunlight back into space, and cirrus cloud thinning (CCT), which aims to allow more long-wave radiation to be emitted from the earth into space.
Climate engineering is generally not well perceived because several techniques for both CDR and SRM have potentially adverse impacts on the environment and elsewhere, which we discuss later. Another major concern raised by critics of climate engineering is that by holding out the prospect of technological ways of avoiding some of the impacts of climate change, it will detract from efforts to reduce emissions (this is known as “mitigation deterrence”).
We need a full portfolio approach to climate response strategies
There are four main strategies for responding to climate change: mitigation (i.e. emission reduction), adaptation to a warmer climate, CDR and SRM. Mitigation and adaptation have been at the heart of most climate change responses, but additional interventions are necessary, as we saw in the first section. We need to think in terms of complementing CO2 emission reduction and adaptation with climate engineering. At a minimum, this should include CDR: we need to deploy techniques to remove CO2 from the atmosphere. Furthermore, despite the risks mentioned in the section on SRM next steps, given the increasing urgency of the climate crisis, we should also learn more about SRM in case its deployment ever becomes unavoidable.
Therefore, the best way of thinking about climate policy is as a portfolio of response strategies, all of which need to be considered, formally included in international and national climate plans, and used where appropriate.
The idea of the portfolio was introduced by Pacala and Sokolow in 2004 in their paper about the concept of ‘stabilisation wedges’. The principle is that we do not know which techniques will work best, so we need to consider all possible options. Then, as knowledge and learning from experience provide information about the most cost-efficient and acceptable techniques, those are prioritised over others. Another approach was developed by McKinsey in 2013 in the form of a global GHG abatement cost curve, where all technologies would be considered, but prioritised according to the estimate of their maximum potential if it were pursued aggressively.
A portfolio of response options including climate engineering are discussed in various research, including the ‘roadmap for rapid decarbonisation’ published in 2017 by Rockström and others, in the US NAS report about solar geoengineering, and in the work of the Carnegie Climate Governance Initiative (C2G).
Figures 1 and 2 illustrate how a portfolio approach can be framed. Figure 1 shows the complementarity of emissions reductions and CDR to meet the Paris climate goal, and is largely adopted in the scientific literature.

However, it is also important to include SRM in the portfolio, which is illustrated in Figure 2.
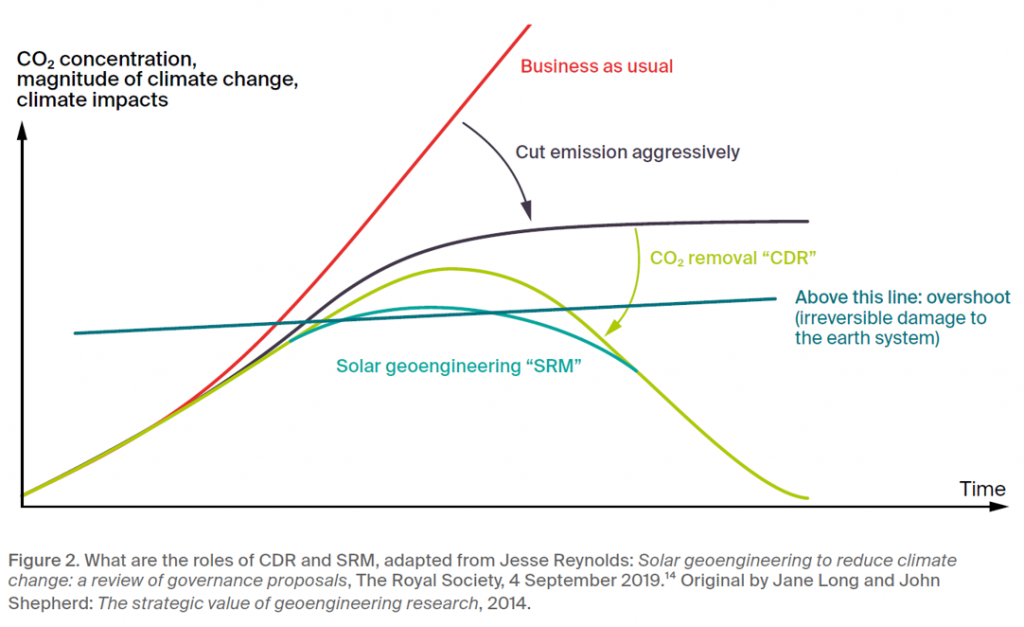
Of the four main response strategies, two address the causes of climate change:
- The underlying anthropogenic cause of climate change can only be addressed by mitigation efforts to reduce emissions of CO2 and other greenhouse gasses. The main strategies for achieving this involve low-carbon energy sources and increased energy conservation. This is highly important and can help achieve a significant share of the change needed to meet the Paris targets. It is essential to reiterate that emissions reduction is the priority and should be intensified. However, on its own, this will not be enough to avoid dangerous consequences from climate change.
- CDR should be seen as a necessary supplementary measure. Every effort should be made to deploy those CDR technologies that are effective, safe, environmentally sustainable, socially and ethically responsible, and economically viable. However, there are many obstacles to be cleared before some large-scale deployment can be carefully decided. CDR encompasses many techniques (see chapter 1 of IRGC’s 2020 report), which are very different from one another. Uncertainties in the size of their possible contribution to CO2 removal are substantial and are complicated by feedback effects. CDR may lead to many possible adverse impacts (see next section). In most of its forms, CDR is expensive, and there are no fully-fledged business models because CDR is only partially included in the Paris Agreement’s system of nationally determined contributions (NDCs).
The other two response strategies address some of the consequences of climate change:
- For regions that are particularly badly hit by climate change, adaptation is absolutely necessary as a coping strategy. It contributes to alleviating some of the local consequences but will not be sufficient in all settings.
- The final element of the climate response portfolio is SRM. It is highly ambiguous and controversial but must be considered because a time may come when it could be necessary. We need to prepare for that eventuality in two ways. We need first to develop an approach to the governance of SRM research and then do serious research, including some field testing. In parallel, we must think about the governance of SRM deployment. SRM should never be conceived as an alternative or standalone strategy. It should only be seen as a supplementary approach that may become necessary to add to the other three. Like CDR, SRM involves a large variety of techniques (see chapter 1 of the IRGC 2020 report) that are very different from one another in their impact and consequences (both risks and benefits). In addition, there are large variations in estimates of how much SRM techniques can contribute to reducing warming on a small, regional or large scale.
CDR next steps: research and careful deployment
We need to intensify efforts around both research and deployment of CDR. We also need to include it in national climate policies. The key challenge is to ensure that the deployment of CDR can be environmentally sustainable, economically viable and socially acceptable. However, this may not be easy for a variety of reasons. First, it is not clear how all the captured CO2 can be permanently stored, particularly if geological sequestration proves to be technically limited. Second, CDR raises difficult trade-offs with other priorities. This is particularly true of nature-based solutions (such as afforestation or reforestation), which can compete with food security or biodiversity and ecosystem conservation. Third, there are questions over CDR financing, given that the benefits will be shared globally and not just by those who invest in it. At present, there is no plausibly profitable business model for funding large-scale CDR, and developing policy roadmaps to effectively promote CDR and DACCS might be useful. Fourth, there are challenges around monitoring and calculating how much CO2 is removed from the atmosphere, to count it against NDCs. Fifth, it is unclear what type of CDR technique will be fully acceptable on a large scale. Finally, some international conventions, particularly the Convention on Biological Diversity and the London Protocol and Convention, have dispositions that restrict some deployment.
Each region in the world has its own capacity with regards to CDR. Some have land available which can be used for forestation, agriculture and soil management, in nature-based CDR solutions where biomass and the soil trap CO2 without threatening food security or biodiversity. Other regions have industrial activities that still produce lots of CO2 and where carbon capture and storage (CCS) can be added. A review of nine OECD countries explores how they integrate or plan to integrate CDR into their climate policy regimes, noting various ways of approaching CDR politically, depending on local conditions. The idea is not new: countries have common yet differentiated responsibilities to combat climate change, in a portfolio approach where all CDR techniques must be considered.
SRM next steps: better understanding of a high-risk strategy
The controversy over SRM stems from a range of significant risks and uncertainties associated with these techniques. There will be direct impact on the environment, as not lowering CO2 concentration has impacts on terrestrial as well as marine ecosystems. SRM could cause adverse impacts in numerous areas, including rainfall patterns and evaporation, biodiversity, other important ecosystems services. Then there are also further concerns around:
- Human welfare and social justice.
- Moral hazard (benefits reaped by those who decide to take the risk of SRM and control it, risks imposed on others)
- Mitigation deterrence, a specific form of moral hazard; or the risk of diverting attention from emission reduction (SAI is sometimes viewed as ‘an easy fix’, or ‘fast, cheap and imperfect’).
- The risk of so-called ‘termination shock’ (if SAI were stopped prematurely, it could cause a rapid and devastating catchup surge in temperatures).
- The risk of unilateral deployment, in particular by populist or authoritarian regimes or even deployment by malevolent actors, and related risks of geopolitical conflict.
These are serious concerns. For these reasons, some argue that we should therefore never countenance using SRM.
On the other hand, however, there have been calls to consider SRM in case of a ‘climate emergency’. The Covid-19 pandemic has taught us that, to be prudent, it is reasonable to prepare for uncertain but dramatic situations. In the case of the climate, this would be where the global temperature increases to a point at which shifts in the climate system would materialise, crossing a dangerous threshold and causing irreversible damage. SRM may be necessary for such circumstances. There would be early warning signs that we are reaching such a tipping point, visible particularly in vulnerable environments. Examples include if global sea levels would rise faster than predicted, or if shifts in biodiversity regimes would be observed, triggered by changes in key indicators, such as mean temperature or CO2 concentration or rain patterns. In response to this kind of early signal of impending catastrophe, we could, after a collective agreement, decide to deploy SRM. IPPC’s forthcoming Sixth Assessment Report will discuss SRM. SRM might help save time by ‘flattening the curve’ (analogy with lock-down measures that helped flatten the contamination curve during the Covid-19 pandemic) or bridge a gap (because, as we said above, it does not address the cause of climate change) but only if boundary conditions are internationally negotiated and if certain indicators are met.
As with any technology that holds both promises and risks, scientists are split on SRM. Some stress that small, controlled and careful experimentation should be permitted (such as with SCoPEx) in order to improve the modelling and understanding of impact and consequences. Others stress the uncertainties and argue for the adoption of a full precautionary approach. Policymakers tend to follow the latter, and the current mainstream approaches for dealing with SRM include (a) focusing only on the governance of research (this was discussed by IRGC in 2010 already), and not discussing the governance of deployment, and (b) considering a moratorium, until more scientific evidence is collected about how to do it and the range of possible consequences.
Given the major concerns about SRM, it is inevitable and sensible that movement is slower than on CDR. International conversations among state actors are starting slowly, building upon initiatives such as those from the Solar Radiation Management Governance Initiative (SRMGI) or the Carnegie Climate Governance Initiative (C2G).
Among the various SRM technique, SAI is the focus of both much interest and controversy. With the combination of new and fast-developing technologies for SAI, high uncertainty, and potential for severe consequences, it is advisable to formalise an approach to its governance that acknowledges the specific features of emerging risks.
Our view is that it is prudent to go further on SRM than policymakers are currently willing to. There is no doubt that SRM is very controversial. It may prove to be a terribly bad idea. It has the potential to lead to scary outcomes, and so it is right to be precautionary. However, our view is that prudence requires us to learn more about SRM so that reliable judgements about it can be made. SRM should not be ruled out entirely. It should be seen as one possible instrument in the portfolio of response strategies, alongside mitigation, adaptation and CDR, and it would be beneficial to continue developing scenarios that could unfold concerning respectively research and experimentation, and deployment.
Conclusion: a matter of trade-offs between risks
There are lots of things that we do not know about climate change and the impacts of CDR and SRM. This reflects the complexity of the climate system and its interactions with other systems, and the difficulty of developing climate models that adequately represent what is predictable and what is not. Scientific uncertainty is pervasive. The field of climate engineering is also marked by ambiguity, with different interpretations of the same scientific evidence, as well as different views on matters of justice, equity and fairness. This combination of complexity, uncertainty and ambiguity makes it difficult for policymakers to take decisions in this domain. One way of freeing up the policymaking process would be to devote time and energy towards inclusive and collaborative processes for identifying, understanding and resolving the complex trade-offs that climate engineering entails. For example, how do we balance the potential benefits on climate change against the potential risks to biodiversity, food and energy? There is no simple solution, but that does not mean that we can ignore the question. More fundamentally, climate engineering presents policymakers with a trade-off between action and inaction: do we remain ignorant about crucial aspects of CDR and SRM because of their potential risks, or do we choose to proceed with careful research, despite the risks, including of moral hazard and reputational damage?
Ignorance must not be part of climate policy. If policymakers adopt a full portfolio approach to all possible response strategies, it will help to prepare us for an uncertain future without delaying decisions. The portfolio approach encourages the flexibility and diversity that global climate policy needs and enables adaptation to national conditions. It provides an overarching framework that can make room for CDR and even SRM in national and international policy, but only after evidence-based deliberation about the circumstances in which the use of CDR and SRM is sufficiently safe and acceptable.
- Read the IRGC report on international governance issues on climate engineering: information for policymakers (2020)
Acknowledgements: The author is grateful to Aengus Collins for his input and would like to thank Granger Morgan and Jonathan Wiener for their reviews of this article.
The views presented in this article are those of the author and are not a consensus judgement by IRGC or its reviewers.
- Carnegie Climate Governance Initiative (C2G). https://www.c2g2.net/
- Florin, M.-V., Rouse, P., Hubert, A.-M., Honegger, M. & Reynolds, J. lnternational governance issues on climate engineering information for policymakers. (2020) https://doi.org/5075/epfl-irgc-277726
- Fountain, H. & Flavelle, C. Test Flight for Sunlight-Blocking Research Is Canceled. The New York Times (2021). https://www.nytimes.com/2021/04/02/climate/solar-geoengineering-block-sunlight.html
- Geoengineering Monitor. Stratospheric Aerosol Injection: Technology Briefing. (2021) https://www.geoengineeringmonitor.org/2021/02/stratospheric_aerosol_injection/
- Grieger, K. D., Felgenhauer, T., Renn, O., Wiener, J. & Borsuk, M. Emerging risk governance for stratospheric aerosol injection as a climate management technology. Syst. Decis. 39, 371–382 (2019). https://doi.org/10.1007/s10669-019-09730-6
- Grisé, M. et al. Climate Control: International Legal Mechanisms for Managing the Geopolitical Risks of Geoengineering. (2021). https://www.rand.org/pubs/perspectives/PEA1133-1.html
- Honegger, M., Poralla, M., Michaelowa, A. & Ahonen, H.-M. Who Is Paying for Carbon Dioxide Removal? Designing Policy Instruments for Mobilizing Negative Emissions Technologies. Front. Clim. 3, (2021). https://doi.org/10.3389/fclim.2021.672996
- Horton, J. B., Keith, D. W. & Honegger, M. Implications of the Paris Agreement for Carbon Dioxide Removal and Solar Geoengineering. (2016). https://www.belfercenter.org/sites/default/files/legacy/files/160700_horton-keith-honegger_vp2.pdf
- Intergovernmental Panel on Climate Change (IPCC). Global Warming of 1.5 oC: An IPCC Special Report. (2018). https://www.ipcc.ch/sr15/
- Intergovernmental Panel on Climate Change (IPCC). Sixth Assessment Report (Forthcoming). https://www.ipcc.ch/assessment-report/ar6/
- International Maritime Organisation. The London Protocol and London Convention: How Global Regulation Can Deal With Climate Change Mitigation Technologies to Protect the Marine Environment. (2019). https://wwwcdn.imo.org/localresources/en/OurWork/Environment/Documents/London%20Protocol%20Climate%20Change%20Leaflet%202019%20_FINAL_online%20version.pdf
- Jee, C. Global sea levels are rising even faster than predicted, warns the UN’s climate committee. MIT Technology Review (2019). https://www.technologyreview.com/2019/09/25/132873/global-sea-levels-are-rising-even-faster-than-predicted-says-uns-climate-committee/
- Keutsch Group of Harvard. SCoPEx: Stratospheric Controlled Perturbation Experiment. https://www.keutschgroup.com/scopex
- Lin, A. C. Does Geoengineering Present a Moral Hazard? Ecol. Law Q. 40, 673-712. (2013) https://www.jstor.org/stable/24113611
- Long, J. C. S. & Shepherd, J. G. The Strategic Value of Geoengineering Research. In Global Environmental Change (ed. Freedman, B.) 757–770 (2014). https://doi.org/10.1007/978-94-007-5784-4_24
- Mahajan, A., Tingley, D. & Wagner, G. Fast, cheap, and imperfect? US public opinion about solar geoengineering. Environ. Polit. 28, 523–543 (2019). https://doi.org/10.1080/09644016.2018.1479101
- McKinsey. Pathways to a low-carbon economy: Version 2 of the global greenhouse gas abatement cost curve. (2013). https://www.mckinsey.com/business-functions/sustainability/our-insights/pathways-to-a-low-carbon-economy
- Meckling, J. & Biber, E. A policy roadmap for negative emissions using direct air capture. Nat. Commun. 12, 2051 (2021). https://doi.org/10.1038/s41467-021-22347-1
- Michaelowa, A. Solar Radiation Modification – A “Silver Bullet” Climate Policy for Populist and Authoritarian Regimes? Glob. Policy 12, 119–128 (2021). https://doi.org/10.1111/1758-5899.12872
- Morgan, M. G. & Ricke, K. Cooling the Earth Through Solar Radiation Management: The need for research and an approach to its governance (2010). https://irgc.org/wp-content/uploads/2019/03/SRM_Opinion_Piece_web.pdf
- National Academies of Sciences, Engineering and Medicine. Climate Intervention Is Not a Replacement for Reducing Carbon Emissions – Proposed Intervention Techniques Not Ready for Wide-Scale Deployment. (2015). https://www.nationalacademies.org/news/2015/02/climate-intervention-is-not-a-replacement-for-reducing-carbon-emissions-proposed-intervention-techniques-not-ready-for-wide-scale-deployment
- National Academies of Sciences, Engineering and Medicine. Negative Emissions Technologies and Reliable Sequestration: A Research Agenda. (2018). https://doi.org/10.17226/25259
- National Academies of Sciences, Engineering and Medicine. Reflecting Sunlight Recommendations for Solar Geoengineering Research and Research Governance Report Release (2021). https://doi.org/10.17226/25762
- Olson, R. Geoengineering for Decision Makers. (2011). https://www.wilsoncenter.org/publication/geoengineering-for-decision-makers
- Pacala, S. & Socolow, R. Stabilization Wedges: Solving the Climate Problem for the Next 50 Years with Current Technologies. Science 305, 968–972 (2004). https://doi.org/10.1126/science.1100103
- Parker, A. & Irvine, P. J. The Risk of Termination Shock From Solar Geoengineering. Earths Future 6, 456–467 (2018). https://doi.org/10.1002/2017EF000735
- Reynolds, J. L. Solar geoengineering to reduce climate change: a review of governance proposals. Proc R Soc A 475 (2019). https://doi.org/10.1098/rspa.2019.0255
- Rockström, J. et al. A roadmap for rapid decarbonization. Science 355, 1269–1271 (2017). https://doi.org/10.1126/science.aah3443
- Royal Society and Royal Academy of Engineering. Greenhouse Gas Removal. (2018). https://royalsociety.org/topics-policy/projects/greenhouse-gas-removal/
- Schenuit, F. et al. Carbon Dioxide Removal Policy in the Making: Assessing Developments in 9 OECD Cases. Front. Clim. 3, (2021). https://doi.org/10.3389/fclim.2021.638805
- Secretariat of the Convention on Biological Diversity. Climate-related Geoengineering and Biodiversity. (2017). https://www.cbd.int/climate/geoengineering/
- Smith, D. M. et al. North Atlantic climate far more predictable than models imply. Nature 583, 796–800 (2020). https://doi.org/10.1038/s41586-020-2525-0
- Solar Radiation Management Governance Initiative. https://www.srmgi.org/
- Stephens, J. C. & Surprise, K. The hidden injustices of advancing solar geoengineering research. Glob. Sustain. 3 (2020). https://doi.org/10.1017/sus.2019.28
- UNEP. The Emissions Gap Report 2017. (2017). https://wedocs.unep.org/bitstream/handle/20.500.11822/22070/EGR_2017.pdf
- UNFCCC. Nationally Determined Contributions (NDCs). (2021). https://unfccc.int/process-and-meetings/the-paris-agreement/nationally-determined-contributions-ndcs/nationally-determined-contributions-ndcs
- Wollersheim, L. The 4 Most Critical Global Warming Deadlines and Tipping Points We Must NEVER Forget or, We Go Extinct. Job One for Humanity (2019). https://www.joboneforhumanity.org/the_4_most_critical_global_warming_deadlines_and_tipping_point_we_must_never_forget