The Laboratory of Advanced Separations (LAS) has been engaged in multidisciplinary research focused on material chemistry and engineering at the Å length-scale to develop high-performance inorganic and hybrid membranes for energy-efficient molecular and ionic separation (see figure below). We focus on engineering ultrathin films, down to the thickness of a single atom (graphene) or unit-cell (MOFs), or built from two-dimensional nanosheets (zeolites) using scaleup-conducive chemistries with high precision in pore size and functionality.
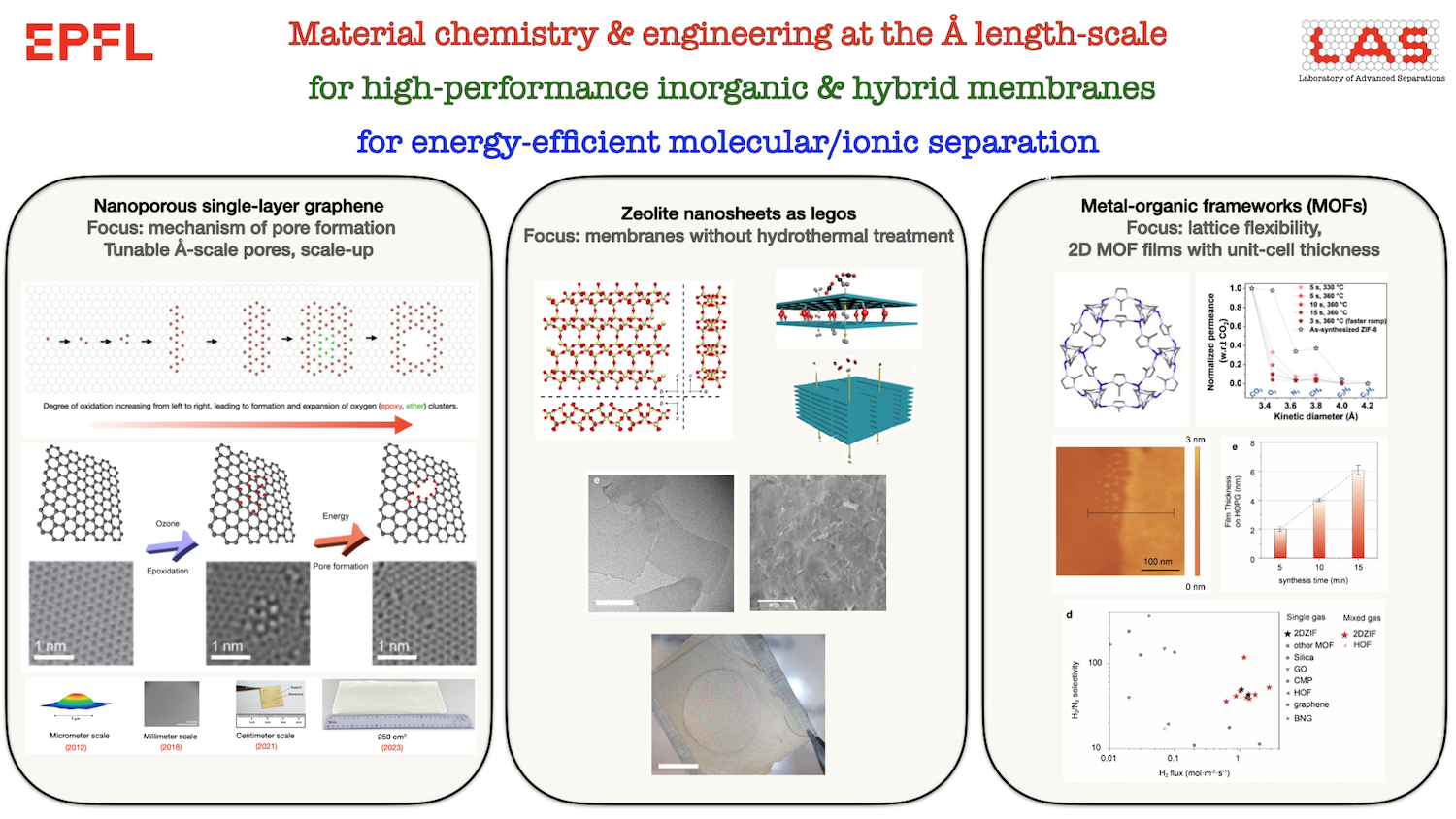
Summary of research direction at the Laboratory of Advanced Separations
space
Our approach is motivated by the fact that porous two-dimensional (2D) films can lead to new regimes in separation beyond the reach of conventional materials such as polymers. This is reflected by the extremely attractive CO2/N2 permeance of atom-thick graphene film hosting Å-scale pores (Science Advances 2021). Our techno-economic analysis for a case study on postcombustion capture identifies that such membranes will cut down the energy and capture penalty, significantly (down to 1 GJ/tonCO2 and 30 $/tonCO2, respectively, Journal of Membrane Science 2021).
Currently, we focus on porous graphene (hosting Å-scale holes), 2D zeolites, and 2D MOFs with application in gas separation. We are aware of the challenges that these novel technologies face in their scale-up and accordingly are also focused on developing chemistry and engineering approaches that are scale-up friendly. Check out our publication, news, as well as twitter (X now) page for recent updates. Check out our openings page for potential position in the group. Feel free to contact Prof. Agrawal at [email protected] if you want to know more about the research @LAS.