Computational design of components for synthetic biology
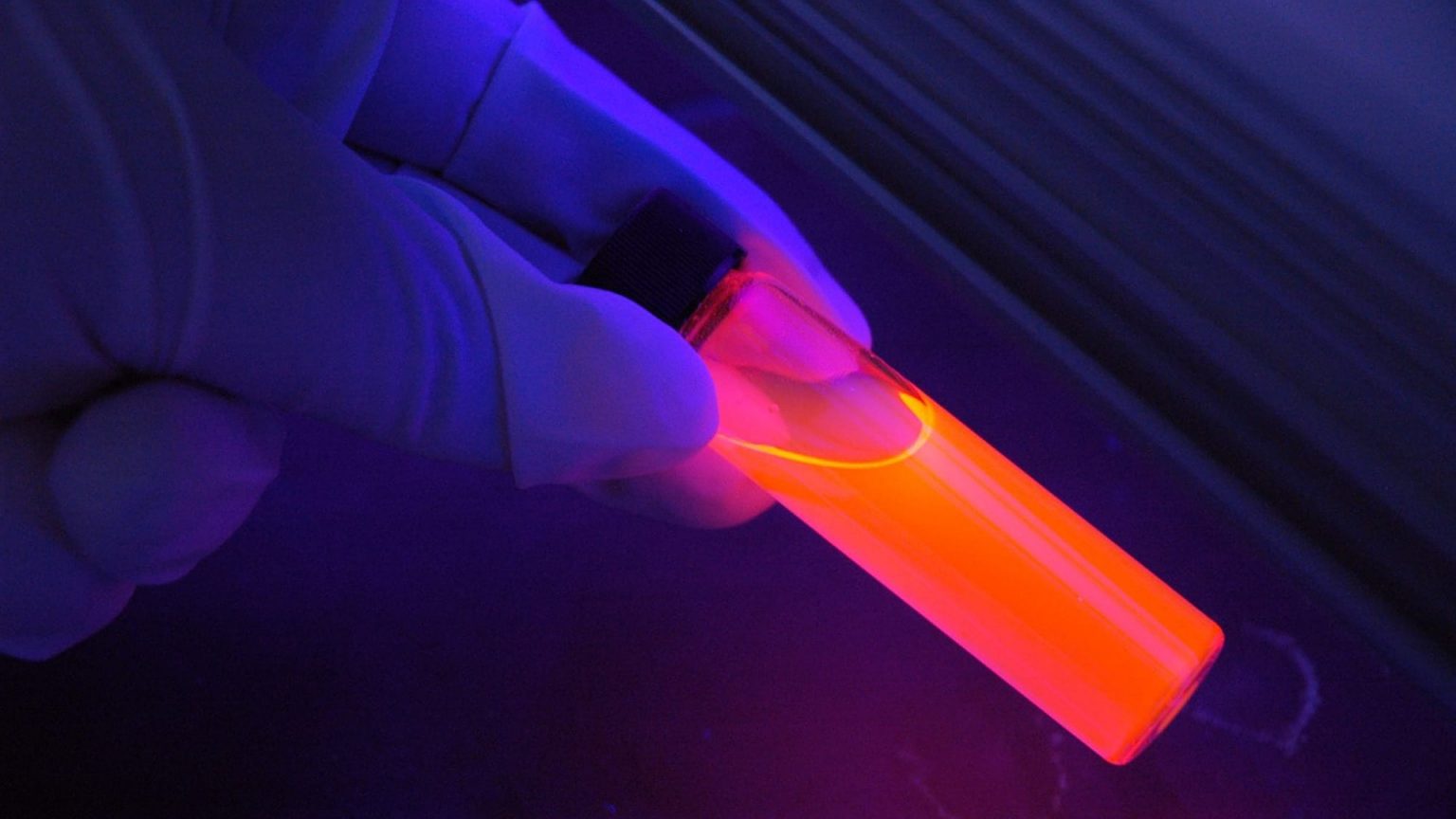
Chemically-controlled protein switches
In synthetic biology, many strategies are pursued to control cell signaling by equipping cells with sophisticated genetic circuits governed by small-molecule-controlled protein switches. However, the repertoire of such protein switches is insufficient for many applications, including those in the translational spaces, where properties such as safety, immunogenicity, drug half-life, and drug side-effects are critical.
Our lab uses computational protein design to repurpose drug-inhibited protein-protein interactions (PPIs) such as OFF- and ON-switches. In OFF-switch systems, the designed binder and drug-receptor form chemically disruptable heterodimers (CDH), which dissociate in the presence of small molecules. In contrast, ON-switches assemble in the presence of a drug via allosteric regulation. The designed switches show robust and precise control over synthetic circuits in mammalian cells.
Our approach effectively expands the chemical space and logic responses in living cells and provides a blueprint to develop new ON- and OFF- switches for basic and translational applications.
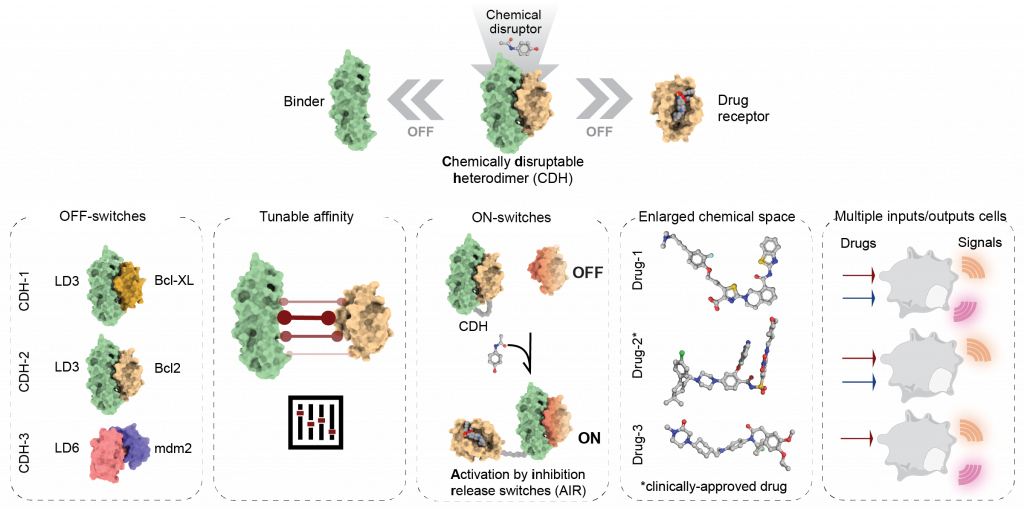
Figure 1. Overview of structure-based design strategies employed to create novel small- molecule responsive switches.
References: Shui S. et al. bioRxiv (2021)
Small-molecule safety switch for T-cell therapy
T-cells can be genetically engineered with chimeric antigen receptors (CARs) to recognize and eliminate cancer cells. However, CAR-T cell therapies can also show off-target effects, resulting in potentially life-threatening situations. To improve the safety of CAR-T-cell therapy, our lab computationally designed a chemically disruptable heterodimer (CDH) incorporated into a synthetic heterodimeric CAR, called STOP-CAR. The CDH self-assembles and can be disrupted by a small-molecule drug. Timed administration of the small-molecule dynamically inactivated the STOP-CAR cells. Our work highlights the potential for structure-based design to add controllable elements to synthetic cellular therapies.
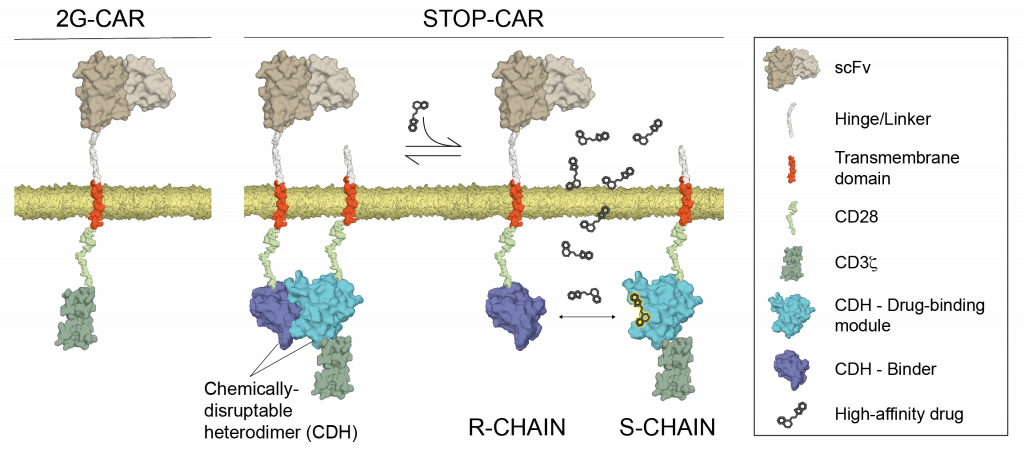
References: Giordano-Attianese G. et al. Nature Biotechnology (2020)
Light-controlled protein switches
Optogenetic control of CRISPR–Cas9 systems has significantly improved our ability to perform genome perturbations in living cells with high precision in time and space. As new Cas orthologues with advantageous properties are rapidly being discovered and engineered, the need for straightforward strategies to control their activity via exogenous stimuli grows. The Cas9 from Neisseria meningitidis (Nme) is a particularly small and target-specific Cas9 orthologue and thus of high interest for in vivo genome editing applications.
In collaboration with the Niopek laboratory, we created the first optogenetic tool to control NmeCas9 activity in mammalian cells via an engineered, light-dependent anti-CRISPR (Acr) protein. These anti-CRISPR proteins potently block NmeCas9 activity in the dark while permitting robust genome editing at various endogenous loci upon blue light irradiation.
Our approach demonstrates optogenetic regulation of a type II-C CRISPR effector and might suggest a new route for the design of optogenetic Acrs.
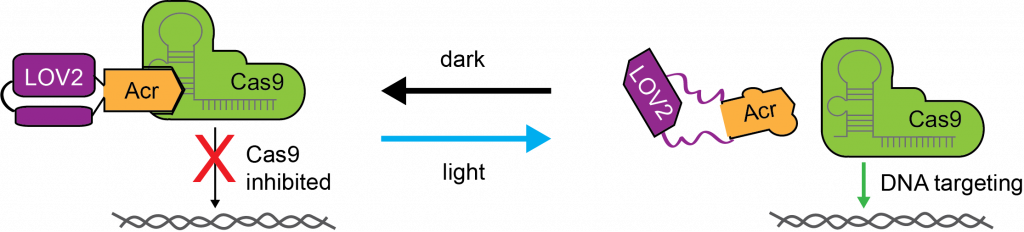
References: Hoffmann M. D. et al. Nucleic Acids Research (2021), Bubeck F. et al. Nature methods (2018)
Design of anti-CRISPR proteins
Acr proteins provide a powerful shut-off mechanism to keep CRISPR-Cas technology in check and enhance the precision at which genome perturbations can be made. However, the available Acr repertoire is limited to naturally occurring variants. To increase the Acrs at our disposal, the design of artificial Acrs is needed. Asa proof-of-concept, our lab used a structure-based design approach on AcrIIC1, a broad-spectrum CRISPR–Cas9 inhibitor, to improve its efficacy on different targets. We converted AcrIIC1 into AcrIIC1X, a potent inhibitor of the Staphylococcus aureus (Sau) Cas9, an orthologue widely used for in vivo genome editing.
Our work introduces designer Acrs as essential biotechnological tools and provides an innovative strategy to safeguard CRISPR technologies.
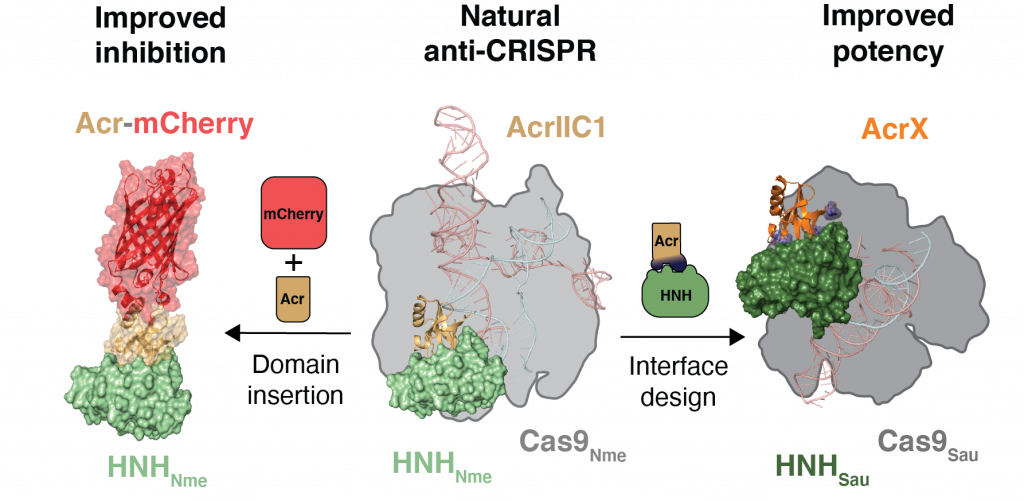
References: Mathony J. et al. Nature chemical biology (2020)