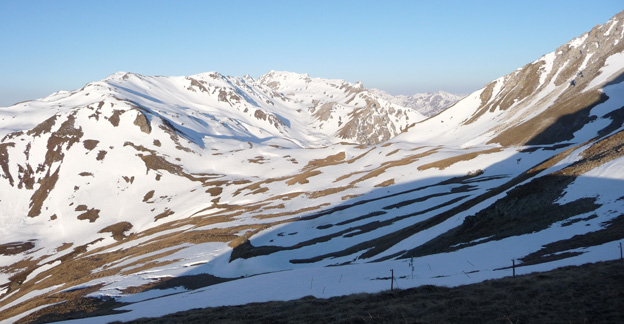
The spatial and temporal dynamics of the mountain snow cover are only incompletely understood. The lack of knowledge on total precipitation amounts in (high) mountains is one important reason for this and has the severe implication that model predictions of high-mountain precipitation as well as changes thereof are not validated and likely to be biased (Grunewald and Lehning 2015). As an additional complication, snow is easily transported by wind and this also changes significantly where snow finally deposits to form the seasonal (or perennial) mountain snow cover. But not only the deposition of snow but also the spatial and temporal variation in melt remains complex. An important feature is the delayed melt of a patchy snow cover in spring (Schlogl, Lehning et al. 2018), where it is observed that snow in depressions resists melt for a long time often creating interesting snow patterns, which are e.g. in Japan interpreted as “Yukigata” (see Figure 1). The melt dynamics are important for a full understanding of streamflow dynamics but also for a correct assessment of effects on the atmosphere, (Mott, Schlogl et al. 2017).
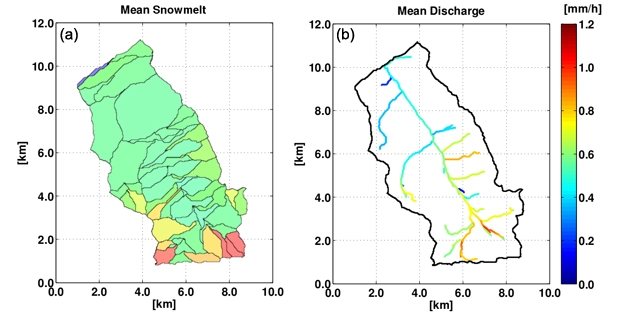
CRYOS investigates both, snow deposition and snow melt in high mountains. An original contribution has been a first investigation of the contribution of flow – particle interactions to spatially varying snow deposition, a process for which the term “preferential deposition” has been coined (Lehning, Lowe et al. 2008). Trying to explain spatial snowfall patterns as a combination of cloud processes (Mott, Scipion et al. 2014, Gerber, Besic et al. 2018), spatially varying deposition (Lehning, Lowe et al. 2008, Dadic, Mott et al. 2010, Mott, Schirmer et al. 2010, Gerber, Mott et al. 2019) and drifting and blowing snow (Zwaaftink, Mott et al. 2013) is a main and primary focus and is achieved by a combination of modelling efforts and laser scanning from airborne (ALS:(Lehning, Gruenewald et al. 2011)) or terrestrial (TLS:(Grunewald, Schirmer et al. 2010)) platforms. With recent progress in the accuracy and of such snow remote sensing, it is now possible to get a good quantitative measure of the spatial amount in snow (snow depth and snow water equivalent). This is exploited to better constrain not only the total snow water storage but also the total (solid) precipitation fields in mountains and in particular the question, how to correctly represent precipitation gradients in larger scale models. With the additional help of numerical modelling such as with Alpine3D (Lehning et al., 2006), it is possible to disentangle the complex processes that shape the mountain snow cover(Vogeli, Lehning et al. 2016). The process research is the basis for applications such as avalanche warning (Schirmer, Lehning et al. 2009).
With respect to melt, it is increasingly realized that a correct description of runoff timing in small catchments depends on the correct description of the spatial melt patterns ((Comola, Schaefli et al. 2015), see Figure 2). Important features of spatial melt are that south slopes melt much faster than other exposures and that the melt period is prolonged by the persistence of snow in depressions (Mott, Gromke et al. 2013) and in avalanche deposits.
References:
Comola, F., B. Schaefli, P. Da Ronco, G. Botter, M. Bavay, A. Rinaldo and M. Lehning (2015). “Scale-dependent effects of solar radiation patterns on the snow-dominated hydrologic response.” Geophysical Research Letters42(10): 3895-3902.
Dadic, R., R. Mott, M. Lehning and P. Burlando (2010). “Wind influence on snow depth distribution and accumulation over glaciers.” Journal of Geophysical Research-Earth Surface115.
Gerber, F., N. Besic, V. Sharma, R. Mott, M. Daniels, M. Gabella, A. Berne, U. Germann and M. Lehning (2018). “Spatial variability in snow precipitation and accumulation in COSMO-WRF simulations and radar estimations over complex terrain.” Cryosphere12(10): 3137-3160.
Gerber, F., R. Mott and M. Lehning (2019). “The Importance of Near-Surface Winter Precipitation Processes in Complex Alpine Terrain.” Journal of Hydrometeorology20(2): 177-196.
Grunewald, T. and M. Lehning (2015). “Are flat-field snow depth measurements representative? A comparison of selected index sites with areal snow depth measurements at the small catchment scale.” Hydrological Processes29(7): 1717-1728.
Grunewald, T., M. Schirmer, R. Mott and M. Lehning (2010). “Spatial and temporal variability of snow depth and ablation rates in a small mountain catchment.” Cryosphere4(2): 215-225.
Lehning, M., T. Gruenewald and M. Schirmer (2011). “Mountain snow distribution governed by an altitudinal gradient and terrain roughness.” Geophysical Research Letters38.
Lehning, M., H. Lowe, M. Ryser and N. Raderschall (2008). “Inhomogeneous precipitation distribution and snow transport in steep terrain.” Water Resources Research44(7).
Lehning, M., I. Volksch, D. Gustafsson, T. A. Nguyen, M. Stahli and M. Zappa (2006). “ALPINE3D: a detailed model of mountain surface processes and its application to snow hydrology.” Hydrological Processes20(10): 2111-2128.
Mott, R., C. Gromke, T. Grunewald and M. Lehning (2013). “Relative importance of advective heat transport and boundary layer decoupling in the melt dynamics of a patchy snow cover.” Advances in Water Resources55: 88-97.
Mott, R., M. Schirmer, M. Bavay, T. Grunewald and M. Lehning (2010). “Understanding snow-transport processes shaping the mountain snow-cover.” Cryosphere4(4): 545-559.
Mott, R., S. Schlogl, L. Dirks and M. Lehning (2017). “Impact of Extreme Land Surface Heterogeneity on Micrometeorology over Spring Snow Cover.” Journal of Hydrometeorology18(10): 2705-2722.
Mott, R., D. Scipion, M. Schneebeli, N. Dawes, A. Berne and M. Lehning (2014). “Orographic effects on snow deposition patterns in mountainous terrain.” Journal of Geophysical Research-Atmospheres119(3): 1419-1439.
Schirmer, M., M. Lehning and J. Schweizer (2009). “Statistical forecasting of regional avalanche danger using simulated snow-cover data.” Journal of Glaciology55(193): 761-768.
Schlogl, S., M. Lehning and R. Mott (2018). “How Are Turbulent Sensible Heat Fluxes and Snow Melt Rates Affected by a Changing Snow Cover Fraction?” Frontiers in Earth Science6.
Vogeli, C., M. Lehning, N. Wever and M. Bavay (2016). “Scaling Precipitation Input to Spatially Distributed Hydrological Models by Measured Snow Distribution.” Frontiers in Earth Science4.
Zwaaftink, C. D. G., H. Lowe, R. Mott, M. Bavay and M. Lehning (2011). “Drifting snow sublimation: A high-resolution 3-D model with temperature and moisture feedbacks.” Journal of Geophysical Research-Atmospheres116.
Zwaaftink, C. D. G., R. Mott and M. Lehning (2013). “Seasonal simulation of drifting snow sublimation in Alpine terrain.” Water Resources Research49(3): 1581-1590.
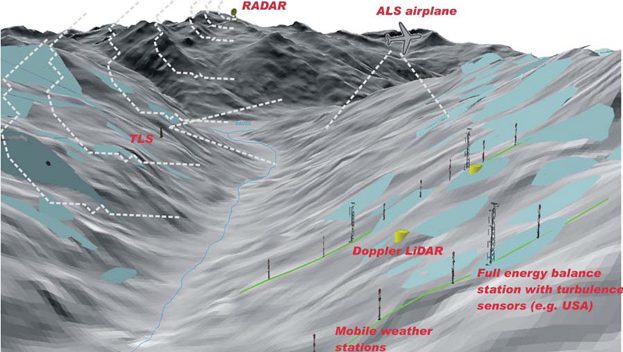
This main SNF research project of Prof. Lehning looks in detail at the snow accumulation processes that happen in mountains. Understanding preferential deposition, snow transport and snow sublimation will help to constrain high-mountain precipitation, which remains elusive even in well equipped countries such as Switzerland. Overall, the project aims at improving our general ability to assess high-mountain water balance, meteorology and natural hazards worldwide. Key information on the project is found on the SNF web page: http://p3.snf.ch/project-179130.