Research Projects

Our research focuses on the understanding
of fundamentals of emergent quantum many-body physics.
Strong correlations in solid-state systems often make the regular
electrons behave differently, and sometimes the resultant
quantum states host quasi-particles that are rather immune
to local environmental disturbance. These quasi-particles are
fundamentally different from electrons or any other fundamental particles. Being fragile, they are elusive and experiments to
detect them are much more challenging; yet their understanding
may change the way we presently look at advanced technology.
Correlated states in few-layers graphene
(Kilian Krötzsch)
The fabrication of graphene-based 2D material heterostructures can lead to the formation of flat bands in the electronic band structure of graphene, significantly enhancing electron-electron correlations that are normally neglected in the single-particle picture used in condensed matter physics. We perform (magneto-)transport measurements on these systems to study a variety of quantum mechanical phenomena: Phases that belong to the large family of quantum Hall effects, which can arise even in the absence of an external magnetic field. This is exemplified by the quantum anomalous Hall effect and in fractional Chern insulators, which result from spontaneous time reversal symmetry breaking. Furthermore, magnetic ordering, spin-valley polarized phases, and superconductivity have been observed in these systems. The stacking order of few-layer graphene plays a crucial role in the formation of these phases, and its properties can be tuned even further. For example by introducing an additional moiré potential or by adding spin-orbit coupling and superconductivity by proximity effects. Consequently, it is a versatile platform for gaining deeper insight into the physics governing strongly correlated systems at cryogenic temperatures.

Correlated states in graphene heterostructures
(Jin Jiang, Zekang Zhou)
Magic angle twisted graphene (MATG) harbors various exotic physical phenomena. The ideas followed by conductance and noise measurements to study the highly correlated topological states, ferromagnetism and also understand the underlying mechanism of superconductivity in the three-layer magic angle twisted graphene (MATTG). Parafermions that obey a non-Ablian state, called zero-energy fractional Majorana fermions, can exist in a system where the quantum Hall effect and superconductivity survive. It is interesting to reveal the parafermions by detecting the Fano factor for shot noise to study the quantum Hall superconductor in the NbN(NbTiN)/Graphene system.
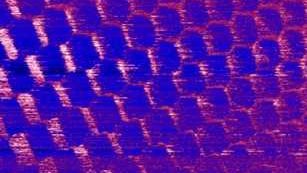
Interferometry in graphene based heterostructures
(Mario Di Luca)
Electrons confined in two-dimensions (2D) under a perpendicular magnetic field at low temperatures give rise to a collective quantum fluid. One phase of such a fluid is known as quantum Hall effect (QHE). Theoretical models followed by experimental evidence have already shown that strong correlations in fractional quantum Hall effect (FQHE) lead to fractionally charged quasiparticles known as anyons. Anyons do not respect fermionic or bosonic exchange statistics, rather exchanging anyons changes the wave function by a phase other than 0 or pi, which differ from state to state. Anyons can be mainly classified into two groups, abelian and non-abelian. The non-abelian anyons are particularly of interest, given their possible application in topological quantum bits. Electron interferometry is an excellent tool to test the intrinsic properties of anyons. The most common interferometer is the Fabry-Perot interferometer (FPI). There are quite few limitations that make observing electron interference in GaAs an arduous task, hence a new platform is required. Graphene, a one-atom-thick layer of carbon atoms, serves as a natural 2D electron gas and it is a perfect substitute platform. It has a unique electronic band structure in which charge carriers behave as massless Dirac fermions with high mobility. In bilayer graphene (BG) , contrary to monolayer, a gap can be opened in the band structure by applying a displacement field, providing a further degree of freedom to control the system.
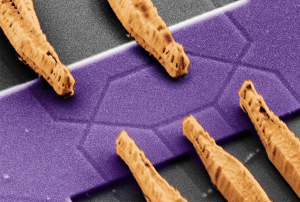
Thermal Hall Conductance of Graphene
(Punam Barman)
Thermal Hall conductance is a universal topological property, which is associated with topological states of matter, mainly in the context of QHE. In this case, equilibrium status of the edge modes are very crucial things to determine topological quantum numbers, which are directly related with conductance (Gs) . By measuring the quantized value of Gs , we can prove that the state of matter is abelian or non – abelian. Now the route is to measure Hall conductance and noise to confirm the presence of counter- propagating modes for 5/2 state in bilayer graphene.
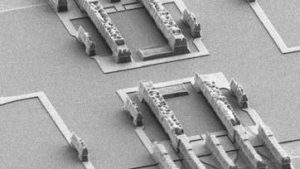
Probing properties of fractional quantum Hall states through entropy measurements
(Emily Hajigeorgiou)
This project is dedicated to exploring the fractional statistics of quasiparticles within the context of the Fractional Quantum Hall Effect, using GaAs quantum dots as the experimental platform. Common methodologies for studying anyonic braiding involve electronic interferometers and anyonic colliders. However, these methods use conductance as the experimental probe, which is not always enough to determine the quantum mechanical ground state of the system. Our approach focuses on measuring entropy, with specific emphasis on quantifying the fractional entropy, which is a signature of nonlocal degrees of freedom such as Majorana zero modes or more exotic non-Abelian anyons.
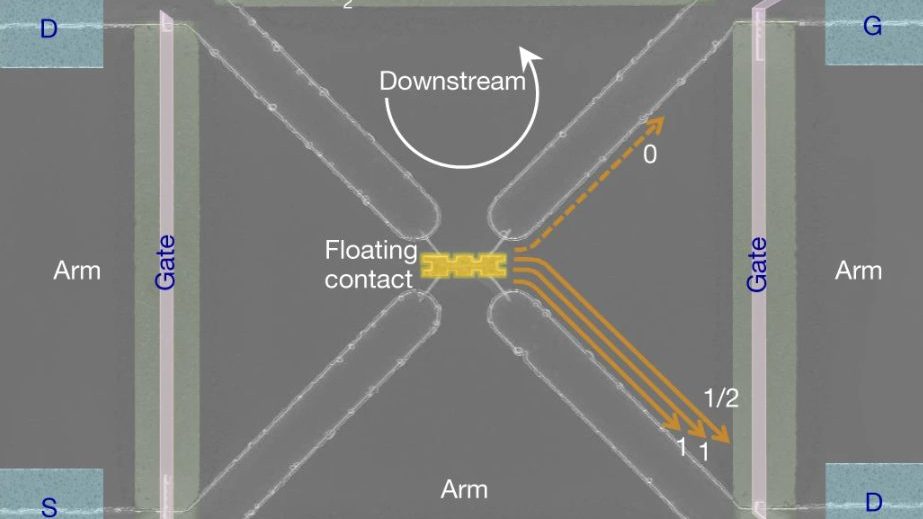