The principle events of the mitotic cell cycle, DNA synthesis, mitosis, and cytokinesis, must be executed with high fidelity and in the correct order if viable progeny cells are to be produced. Cytokinesis requires the cell to solve a spatial problem (to divide in the correct place, usually orthogonally to the plane of chromosome segregation) and a temporal problem (to coordinate cytokinesis with mitosis). Changes in chromosome number resulting from errors of cytokinesis may cause cell death, or alter a cell’s comportment. Asymmetric cytokinesis, which permits the generation of two daughter cells that differ in their shape, size and properties, is important both during development, and for cellular homeostasis in multicellular organisms.
Simple model systems, such as the fission yeast Schizosaccharomyces pombe, provide a powerful tool to study conserved essential processes, such as cell division. S. pombe divides by binary fission after formation of contractile actomyosin ring (CAR), which contracts at the end of mitosis, guiding deposition of new membranes and cell wall material to divide the cell by formation of a new cell wall, or division septum. The Septation Initiation Network (SIN) is a signal transduction network that is essential for cytokinesis. Failure of SIN signalling leads to defects in CAR assembly, stabilisation and contraction, resulting in multinucleated cells, as growth and nuclear division continue. If the SIN is activated but cannot be turned off, it locks the cell into a cytokinesis “subcycle”, where septa are repeatedly synthesised but not cleaved. If the SIN is activated outwith mitosis, then cytokinesis is triggered independent of cell cycle position, breaking the normal dependency of cytokinesis upon exit from mitosis. The SIN is also essential in meiosis to regulate the deposition of membranes at the end of the meiotic cell cycle as the individual nuclei are converted into spores. The regulation of the SIN is different in meiosis (Krapp et al 2014); we are investigating the underlying mechanisms to understand why this is so.
Elements of the SIN have been conserved through evolution, in the form of the Mitotic Exit Network in budding yeast, or the HIPPO pathway in multicellular organisms. The SIN is also conserved in many pathogenic fungi, such as Pneumocystis, that are problematic in a clinical setting where patients are immunocompromised. Study of SIN signalling in a closely related, non-pathogenic organism such as S. pombe may provide insights that open new treatment possibilities.
Genetic screens have identified 13 essential genes required for SIN signalling, and multiple non-essential regulators that contribute to the fidelity of cytokinesis (reviewed by Simanis, 2015). Figure 1 (below) shows how these gene products are thought to interact.
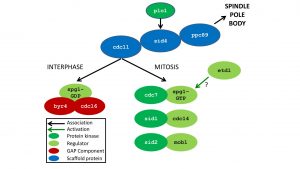
The Figure shows the 13 essential components of the SIN, and the regulators DMA1p. The arrow from cdc11 shows proteins associatednwith the SPB in interphase or mitosis
Components of the SIN
Cytokinesis requires intact spindle pole bodies (SPBs). All the essential core SIN proteins, except Etd1p, are located at the SPB via a tripartite scaffold. Ppc89p acts as an anchor for the other SIN scaffold proteins. Ppc89 is essential for Sid4p localisation to the SPB, but not vice-versa. Sid4p is essential for Cdc11p association to the SPB, but not vice-versa, giving the hierarchical order shown. The SIN plays multiple roles in cytokinesis and meiosis (see Simanis, 2015, for review).
Spg1p is a signalling GTPase that can induce cytokinesis from any point of the cell cycle, if activated. Its principle regulator is a GAP (Cdc16p) with which it interacts through a scaffold (Byr4p). The core of the SIN is three protein kinases (Cdc7p, Sid1p and Sid2p, and their regulators; see figure 1). The SIN also requires CDK1 and the POLO kinase homologue Plo1p for signalling.
SIN signalling initiates from the cytoplasmic face of the SPB. Localisation of SIN proteins to the SPB is important for their function, and each protein kinase has a unique pattern of association with the SPB during mitosis (reviewed by Simanis, 2015). Perturbation of SIN protein localisation is associated with deregulated signalling.
Our research focusses upon the rate-limiting steps of the SIN, which are the focal points for regulation of its signalling in mitosis and meiosis. We have used classical and molecular genetic techniques to generate conditional alleles of SIN genes. We have taken three approaches:
1. dominant negative mutants, which interfere with the function of the wildtype allele
2. low-fidelity PCR amplification followed by replacement of the wild-type allele to generate novel alleles selecting for sensitivity to changes in temperature, or alteration of the composition of the growth medium.
3. directed suppressor screens, based on the techniques used to isolate bypass suppressors of the essential GAP, cdc16 (Fournier et al., 2000).
These have yielded separation-of-function mutant alleles of several SIN genes, where the defect lies in a single functional domain. Targetted suppressor screens using such mutants as a starting point has revealed novel genetic interactions within the SIN. The effects of
these mutants upon cell division and meiosis is being analysed. We are studying the localisation of SIN proteins in these mutants, and their effects on the activity of the signalling GTPase spg1p, and the three core SIN kinases.
Our research focusses upon the rate-limiting steps of the SIN, which are the focal points for regulation of its signalling in mitosis and meiosis. We have used classical and molecular genetic techniques to generate conditional alleles of SIN genes. We have taken three approaches:
1. dominant negative mutants, which interfere with the function of the wildtype allele
2. low-fidelity PCR amplification followed by replacement of the wild-type allele to generate novel alleles selecting for sensitivity to changes in temperature, or alteration of the composition of the growth medium.
3. directed suppressor screens, based on the techniques used to isolate bypass suppressors of the essential GAP, cdc16 (Fournier et al., 2000).
These have yielded separation-of-function mutant alleles of several SIN genes, where the defect lies in a single functional domain. Targetted suppressor screens using such mutants as a starting point has revealed novel genetic interactions within the SIN. The effects of
these mutants upon cell division and meiosis is being analysed. We are studying the localisation of SIN proteins in these mutants, and their effects on the activity of the signalling GTPase spg1p, and the three core SIN kinases.
The SIN is essential for spore formation at the end of meiosis (Krapp et al 2006). We used SILAC labelling to study the relative levels of almost 3300 S. pombe proteins during meiosis (Krapp et al., 2019). We observed a high degree of post-transcriptional regulation of protein level during meiosis, and a global switch from anabolic to catabolic processes. Correlation with previous datasets revealed that some mRNAs persist, untranslated, after the protein decays during meiosis, while some stable proteins persist after their corresponding mRNA decays. We also uncovered a role for the ESCRT-III complex in nuclear division and spore formation (Krapp et al., 2019)
The ubiquitin ligase dma1p is required to prevent SIN signalling in cells arrested in mitosis. In meiosis it is required for spore formation. In contrast to loss of SIN function, which blocks spore formation, 75% of asci from a dma1-null mutant meiosis contain ≤3 spores, despite completing both meiotic divisions (see dmameiosis figure). We found that three SIN proteins (cdc7p, sid4p and cdc11p) are degraded at the end of meiosis, dependent upon dma1p function. A candidate approach revealed that dma1p also has other meiotic targets, but these do not explain the failure to form the correct number of spores (Krapp et al,.2014). To identify other proteins whose elimination depends on dma1p, we have examined the relative levels of proteins during meiosis in dma1-D diploids, and compared it to our published wild-type dataset. The relative level of a small number of proteins changes differently compared to wild-type; we are examining the meiotic role of these proteins.
Our research focusses upon the rate-limiting steps of the SIN, which are the focal points for regulation of its signalling in mitosis and meiosis. We have used classical and molecular genetic techniques to generate conditional alleles of SIN genes. We have taken three approaches:
1. dominant negative mutants, which interfere with the function of the wildtype allele
2. low-fidelity PCR amplification followed by replacement of the wild-type allele to generate novel alleles selecting for sensitivity to changes in temperature, or alteration of the composition of the growth medium.
3. directed suppressor screens, based on the techniques used to isolate bypass suppressors of the essential GAP, cdc16 (Fournier et al., 2000).
These have yielded separation-of-function mutant alleles of several SIN genes, where the defect lies in a single functional domain. Targetted suppressor screens using such mutants as a starting point has revealed novel genetic interactions within the SIN. The effects of
these mutants upon cell division and meiosis is being analysed. We are studying the localisation of SIN proteins in these mutants, and their effects on the activity of the signalling GTPase spg1p, and the three core SIN kinases.
Krapp A, Hamelin R, Armand F, Chiappe D, Krapp L, Cano E, Moniatte M, Simanis V.
Cell Reports. 26 1044-1058
Simanis V. Journal of Cell Science, 128, 1465-1474.
Dma1-dependent degradation of SIN proteins during meiosis in Schizosaccharomyces pombe. (2014)
Krapp A, Simanis V.
Journal of Cell Science 127, 3149-3161.
The role of Schizosaccharomyces pombe dma1 in spore formation during meiosis. (2010)
Krapp A., Cano Del Rosario E., and Simanis V.
Journal of Cell Science. 123 3284 – 3293.
Krapp A, Collin P, Cokoja A, Dischinger S, Cano E, Simanis V.
Journal of Cell Science 119, 2882-2891.
Fournier N., Cerutti, L., Beltraminelli, N., Salimova, E. and Simanis, V.
Archives of Microbiology, 175, 62 – 69.